This article has been reviewed according to Science X's editorial process and policies. Editors have highlighted the following attributes while ensuring the content's credibility:
fact-checked
trusted source
proofread
From organics to fuels: Computational models can accelerate and scale up biomass conversion
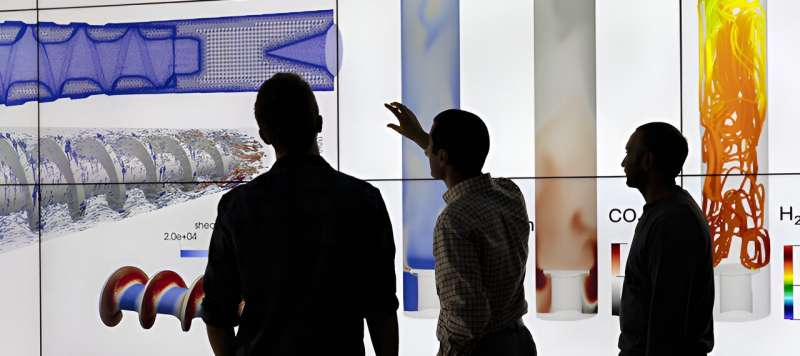
Your yard clippings could eventually fuel an airplane. National Renewable Energy Laboratory (NREL) researchers are tackling the difficult task of using agricultural waste generated from plant-to-food processing to make fuels that can power an airplane or a ship rather than relying on fossil fuels.
Researchers in NREL's Computational Science Center are hard at work enabling the conversion of biomass to fuels and products at the industrial scale. Computational tools can model biomass conversion with domain sizes ranging from small lab beakers to factory scale, which can help accelerate and de-risk the newest technology developments.
Recent computational studies in biomass conversion have made use of funding from the U.S. Department of Energy's Bioenergy Technologies Office (BETO) and the high-performance computing resources at NREL to investigate and provide solutions for complex conversion challenges, like making sustainable aviation fuels (SAF).
Computational modeling can provide the added benefit of enabling scientists and engineers to test systems without the risk of expensive equipment that may not work as intended. This simulation capability can save companies millions of dollars by avoiding equipment upsets and failures.
The turn of the screw feeder
Lignocellulosic biomass (e.g., pine residue, corn stover/cobs), is an abundant raw material that has drawn considerable interest from researchers as a biofuel feedstock. One of the first steps in biomass conversion is the handling of this feedstock material, which is highly variable in terms of particle size, ash, and moisture content.
The conversion process begins by feeding solids into a pressurized or high-temperature reactor for breaking down the biopolymers. Predicting the energy requirements and mechanical stresses on the equipment for this feeding process is critical, as it governs the operation of the entire biomass conversion plant.
That is where computational science can help. NREL research scientist Mohammad Rahimi and co-researchers, working as part of the Feedstock-Conversion Interface Consortium (FCIC) funded by BETO, developed a predictive computational model for biomass screw feeders that captures the viscous, non-Newtonian, and compressible behavior of biomass slurries. The model successfully predicts the location of a biomass plug, which can help refinery operators identify and remedy it.
"Mechanical failure in screw feeders is one of the main reasons for unpredictable downtimes for industrial biomass processing plants," Rahimi said. "Avoiding such failures can significantly improve the profitability and production performance of these industries. We developed a computational model that can enable these industries to identify optimal operating conditions for their biomass conveying systems and prevent most of their future mechanical failures."
A pathway to industrial-scale sugar conversion to BDO
Much like the prediction of mechanical failure and energy demands in the conversion process, computational tools can address the problem of controlling oxygen distribution in industrial-scale bioreactors producing fuels from biomass-derived sugars. One of the promising pathways is the production of 2,3-butanediol (BDO), an important intermediate for downstream production of SAFs and chemical products.
Zymomonas mobilis, a microbe, has shown great promise at the lab scale in converting sugars to BDO, but a controlled microaerated environment is crucial to achieve high BDO yields, creating a problem for the scale-up for Zymomonas-assisted BDO synthesis. New BETO-funded research led by NREL scientist Hari Sitaraman and team, published in Chemical Engineering Research and Design, uses a high-fidelity computational model for reacting multiphase flows in large-scale bioreactors to address this scale-up challenge.
The researchers studied BDO production in industrial microaerated bioreactors using a coupled bioreaction and computational fluid dynamics model. The study optimized aeration rate and reactor geometry to improve BDO yield by 25% at the 500-million-liter scale and showed that traditional bubble column reactors for ethanol production may need some design changes before repurposing it for BDO production. This study will be crucial for BDO production scale-up and will be of interest to industry (e.g., a current partnership between Bioprincipia and NREL).
The total (virtual biorefinery) package
As computational tools are used to simulate the individual steps of biomass conversion, it makes sense that researchers may utilize computational methods to link these simulations and build a virtual biorefinery capturing the entire process of biomass conversion from feedstock to fuel. Recent BETO-funded work from Ethan Young, NREL computational science researcher, and team demonstrates a virtual engineering (VE) software to accelerate development and reduce risk for market-relevant biomass conversion processes.
The BETO-funded team created multiphysics and multiscale models, and they are developing a proof-of-concept software infrastructure with future work to enable use in production. The motivation behind the VE project is that, while standalone simulations and models are necessary for accurately predicting the fine-grained interaction physics of a single chemical process, an end-to-end simulation in which the linked outcomes of process one affect process two (and so on) is necessary to predict the overall performance of a bioconversion plant.
The VE software provides a user interface and library of tools to enable these intermodel connections to be easily defined and modified to predict the effect of prescribed conditions or to design an optimal control strategy. The computational models for each conversion step (e.g., pretreatment or hydrolysis) in the VE framework have been previously developed and validated by the team.
The VE software is freely available on GitHub. The framework is currently designed for biochemical conversion but can be extended to new domains such as thermochemical conversion and with other feedstocks (e.g., plastics, municipal solid waste).
"Linking these different simulations together lets you consider a complete cascade of dependencies and provides a clearer picture of how design and operating changes will affect conversion outcomes in the context of a complete biorefinery," Young said. "Testing out different hardware choices and conversion pathways using this linked computational approach is faster and less expensive relative to an experimental approach, which we believe will accelerate new developments in biomass."
More information: Hariswaran Sitaraman et al, A reacting multiphase computational flow model for 2,3-butanediol synthesis in industrial-scale bioreactors, Chemical Engineering Research and Design (2023). DOI: 10.1016/j.cherd.2023.07.031