This article has been reviewed according to Science X's editorial process and policies. Editors have highlighted the following attributes while ensuring the content's credibility:
fact-checked
peer-reviewed publication
trusted source
proofread
Researchers characterize how passivation layer forms in lithium-ion batteries
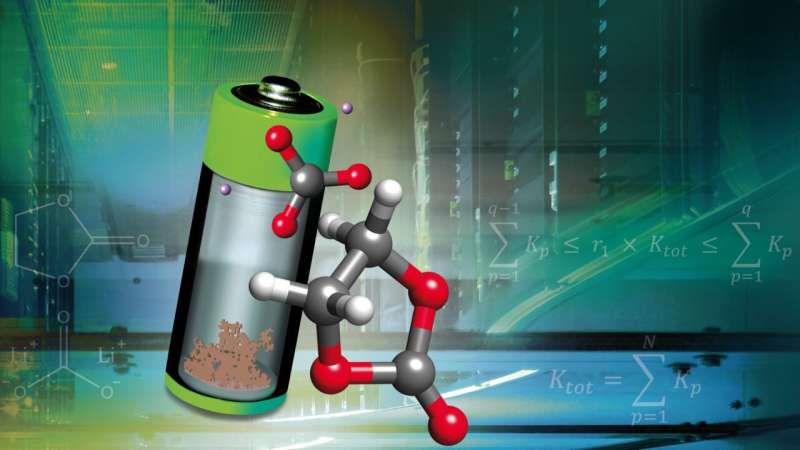
In our daily lives, lithium-ion batteries have become indispensable. They function only because of a passivation layer that forms during their initial cycle. As researchers at Karlsruhe Institute of Technology (KIT) found out via simulations, this solid electrolyte interphase develops not directly at the electrode but aggregates in the solution. Their study has been published in the journal Advanced Energy Materials. Their findings allow the optimization of the performance and lifetime of future batteries.
From smartphones to electric cars—wherever a mobile energy source is required—it is almost always a lithium-ion battery that does the job. An essential part of the reliable function of this and other liquid electrolyte batteries is the solid electrolyte interphase (SEI). This passivation layer forms when voltage is applied for the first time. The electrolyte is being decomposed in the immediate vicinity of the surface. Until now, it remained unclear how the particles in the electrolytes form a layer that is up to 100 nanometers thick on the surface of the electrode since the decomposition reaction is only possible within a few nanometers distance from the surface.
The passivation layer on the anode surface is crucial to the electrochemical capacity and lifetime of a lithium-ion battery because it is highly stressed with every charging cycle. When the SEI is broken up during this process, the electrolyte is further decomposed and the battery's capacity is reduced—a process that determines the lifetime of a battery. With the right knowledge of the SEI's growth and composition, the properties of a battery can be controlled. But so far, no experimental or computer-aided approach was sufficient to decipher the SEI's complex growth processes that take place on a very wide scale and in different dimensions.
Researchers at the KIT Institute of Nanotechnology (INT) have now managed to characterize the formation of the SEI with a multi-scale approach. "This solves one of the great mysteries regarding an essential part of all liquid electrolyte batteries—especially the lithium-ion batteries we all use every day," says Professor Wolfgang Wenzel, director of the research group "Multiscale Materials Modelling and Virtual Design" at INT, which is involved in the large-scale European research initiative BATTERY 2030+ that aims to develop safe, affordable, long-lasting, sustainable high-performance batteries for the future.
More than 50,000 simulations for different reaction conditions
To examine the growth and composition of the passivation layer at the anode of liquid electrolyte batteries, the researchers at INT generated an ensemble of more than 50,000 simulations representing different reaction conditions. They found that the growth of the organic SEI follows a solution-mediated pathway. First, SEI precursors that are formed directly at the surface join far away from the electrode surface via a nucleation process. The subsequent rapid growth of the nuclei leads to the formation of a porous layer that eventually covers the electrode surface.
These findings offer a solution to the paradoxical situation that SEI constituents can form only near the surface, where electrons are available, but their growth would stop once this narrow region is covered. "We were able to identify the key reaction parameters that determine SEI thickness," explains Dr. Saibal Jana, postdoc at INT and one of the authors of the study.
"This will enable the future development of electrolytes and suitable additives that control the properties of the SEI and optimize the battery's performance and lifetime."
More information: Meysam Esmaeilpour et al, A Solution‐Mediated Pathway for the Growth of the Solid Electrolyte Interphase in Lithium‐Ion Batteries, Advanced Energy Materials (2023). DOI: 10.1002/aenm.202203966